Note: This 1998 article was written around the DL660 module, which was common at the time. Today we have several other types of modules, but with similar characteristics. The explanations given in this article apply to these more modern modules.
LASER is light, but light of a different nature. To understand how a LASER diode works readers must have some sense of the type of radiation that is obtained from a LASER and how it is produced.
Thus, we will initially see how the LASER works in a general way to then talk about semiconductor LASERS or LASER diodes.
LASER stands for Light Amplification by Stimulated Emission of Radiation.
As the name suggests, LASER is nothing more than a device that amplifies the light emitting it with characteristics that we cannot get from common sources like lamps, LEDs, etc.
HOW IT WORKS
For didactic purposes we will explain how a LASER works by making as an example the traditional type which uses a ruby ??stick and that can be represented in a simplified form, as we observed in Figure 1.
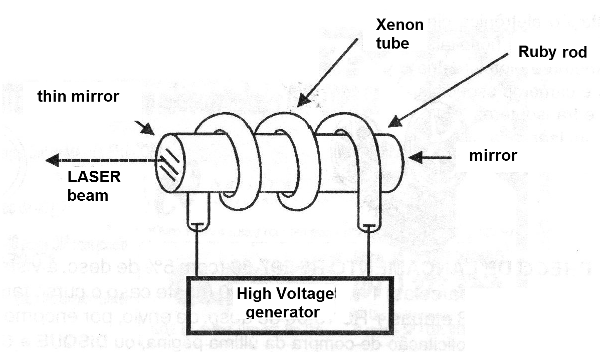
In this arrangement a cylindrical ruby ??rod has two mirrored faces, one being thicker than the other. The mirrored side is the inner one so that light or radiation that is produced internally to the device undergoes reflections in these mirrors.
Around the ruby ??stick and rolled up a xenon tube, a powerful photographic flash lamp that is powered by a high voltage discharge circuit.
When a high voltage discharge is produced in the flash tube, it emits a strong pulse of light that is absorbed by the atoms of the ruby ??stick. This absorption occurs by the electrons around the atoms of the material (which consists of an aluminum oxide), which leave their normal orbits and pass to orbits with higher energy levels, see Figure 2.
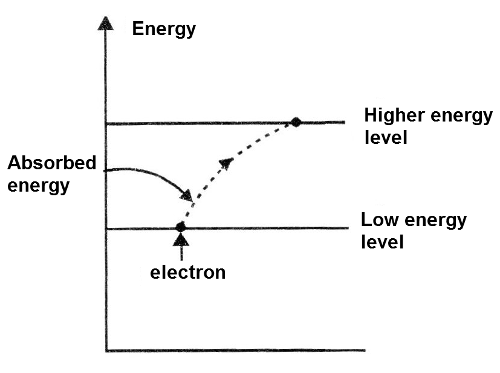
The pulse of light must be extremely strong so that most of the ruby ??atoms have their electrons brought to the higher energy levels, i.o., there is a "population inversion".
The population of the electrons that are in the normal energy level happens to be smaller than the population of the electrons that are in the highest level of energy. This condition is fundamental to obtaining the LASER effect. However, electrons can not stay indefinitely at higher energy levels by retaining absorbed energy.
A fraction of a second later to the absorbed energy, the first electrons return to their original level of energy and in this process they return the energy absorbed.
According to the distance between the original level and the level at which the electrons were, we have the amount of energy returned in the process and therefore the wavelength of the radiation emitted, Figure 3.
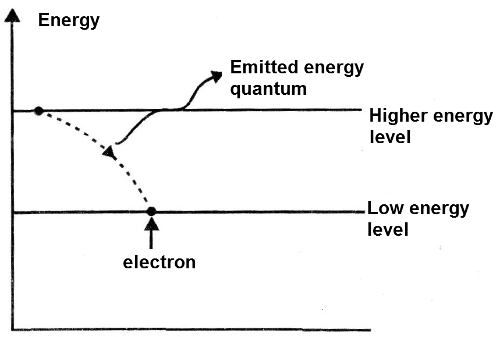
The photon of energy, which can be the visible light or in another frequency of the spectrum, but always of single frequency, has the effect of stimulating the other electrons of the material that are at the highest level of energy to also "return" the absorbed energy.
Thus, by "slamming" on a nearby electron, the photon causes the return of a new photon and we have two photons emitted within the material.
Two photons can "hit" on two new electrons, forcing them to lower their energy level and thus the emission of two more photons occurs. The four photons produce eight, and this in a very fast chain process, see Figure 4.
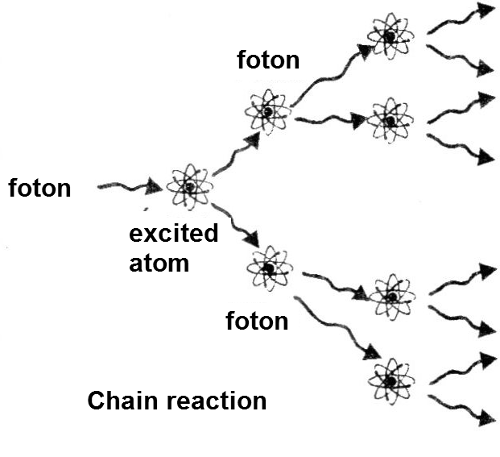
The photons that tend to escape the sides of the material do not do so initially, going back inside the material in order to accelerate the process, because the ruby sides are mirrored. The effect is explosive and in a fraction of a second all electrons are forced to return the absorbed energy in an almost synchronized way and all emitting photons with the same frequency.
The concentration of this energy is such that the mirroring can no longer retain the beam of photons produced and it "escapes" the thinnest side in the form of a narrowed beam of radiation, concentrated and monochromatic, Figure 5.
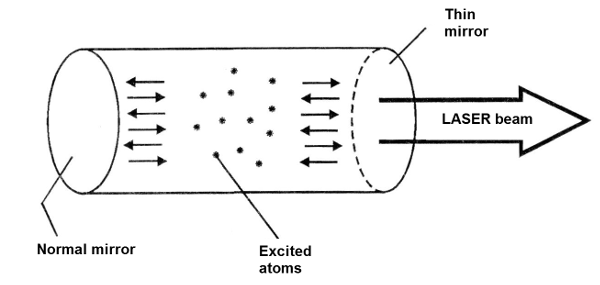
This is the LASER beam which has special characteristics that differentiate its radiation from ordinary light.
In the example we gave, we have a pulsating LASER, since the emission must be done with the production of interval pulses of the flash so that the electrons absorb and return the energy.
However, it is possible to obtain the same effect continuously in gas and injection LASERS, but the principle of operation is the same.
A DIFFERENT RADIATION
The radiation emitted is light and its color depends on the wavelength or energy of the photons.
In the case of ruby ??this radiation is in the visible spectrum, appearing in the form of a thin beam of red light. However, the radiation emitted by an LASER has its own characteristics that differentiate it from ordinary light, let's see:
A) Monochromatic
The "jumps" of the electrons in the return of the energy occur between well defined levels of energy. This means that the radiation emitted has a unique frequency that determines the color of the light, if it is in the visible spectrum.
Of course, the radiation can also be in the infrared and even the ultraviolet range, depending only on the characteristics of the material used.
The most common LASERS today are those operating in the infrared and visible range between red and green.
Thus, unlike an incandescent lamp which has a broad spectrum in where radiation of many wavelengths or colors are mixed, LASER radiation has a narrow spectrum, Figure 6.
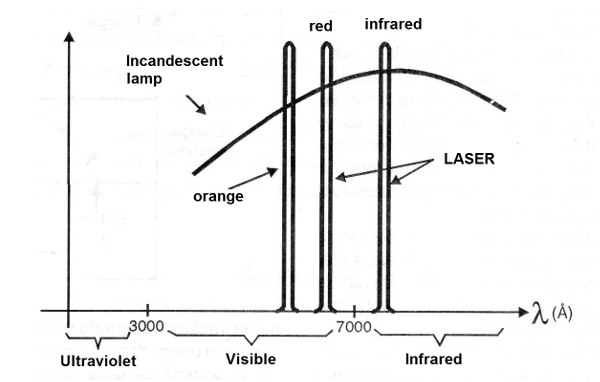
We can compare a common lamp to a circuit that generates a noise filling an entire spectrum band and the LASER to a synchronized circuit that emits single frequency signals.
B) Consistent
LASERS are normally specified as coherent sources of light, i.e., those in which the radiation is emitted in a very narrow beam with a regular phase and a very narrow frequency range.
In practice, however, this does not occur, and most common LASERS only approximate what would be an ideal coherent source. In any case, even if it is not an absolutely coherent source, an LASER can be considered as such when compared to other common sources of radiation.
C) Concentrated
In addition to the laser beam being emitted in a very narrow beam, i.e., with a very small opening, it concentrates a lot of energy.
Thus, if the amount of energy absorbed in one second (duration of the driven flash pulse) is 1 watt, if the subsequent LASER emission process lasts 1 millionth of a second, it will correspond to an instantaneous power of 1 million of watts. Divide the time by 1 million to multiply the power by 1 million.
This explains why the LASER beam can pierce a sheet of steel.
A beam which continuously concentrates such power and on a very small surface generates enough heat to vaporize a hard material such as iron. It is clear that most common LASERS, especially household types and electronic device ones, have wattages that are measured in fractions of watts and therefore can not have considerable destructive effects.
However, energy concentrated in a very narrow beam can have detrimental effects on vision. Sensitive cells in our eye can be destroyed if we directly observe a LASER beam.
The label of "danger" that accompanies any device emitting an LASER radiation is not a joke. (Figure 7)
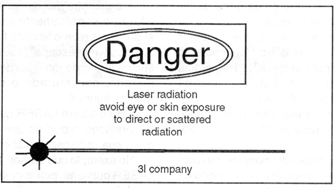
Myth
Many people think it is possible to observe a LASER beam sideways, i.e., that it is visible as a thin line leaving the source. However, it is only possible to observe it if there is light in suspended particles (dust, smoke, etc.) Figure 8.
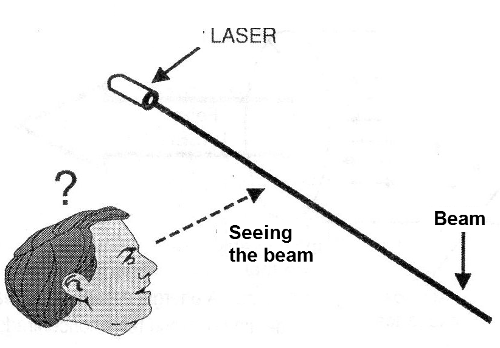
SEMICONDUCTOR LASER
Semiconductor technology enables the manufacture of LASERS of reduced dimensions from a structure reminiscent of LEDS.
In fact, if we consider that an LED is a source of monochromatic light, we can start from this point to change its basic structure and achieve coherent and concentrated light.
Semiconductor LASERS are a reality nowadays and the basis of many electronic devices such as CD players, videodiscs and CD-ROM players.
In fact, it is only with the use of semiconductor LASERS that such devices have become practical and compact enough to allow mass use.
In Figure 9 we have an example of a semiconductor injection LASER that can be used in several practical devices.
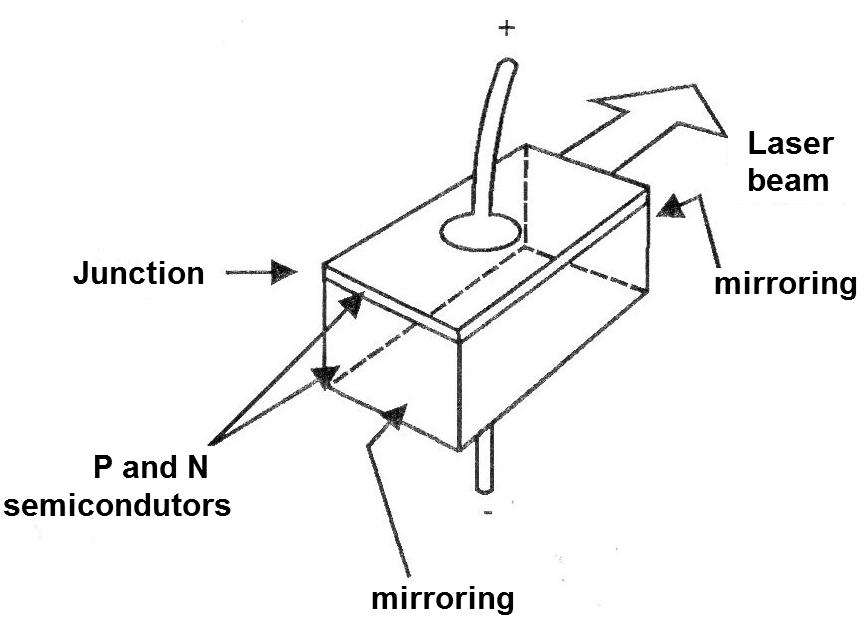
Materials such as gallium arsenide (GaAs) doped with several substances allow to obtain LASERS with wavelengths ranging from infrared to the most part of the visible spectrum.
The operating principle of a semiconductor LASER with the basic structure shown in Figure 9 is as follows:
If the device, which has the characteristics of a diode, is polarized with a low current, the driving occurs at a low level and the light emission will be made in a random manner. The device behaves like a simple LED.
However, if the device is driven by an intense current, the atoms of the material are driven enough that a population inversion occurs, that is, more electrons appear at higher levels of energy than at normal levels.
When the electrons in higher energy levels return to the normal level, there is a stimulated emission of energy and thus the production of the radiation that characterizes the LASER.
This emission is favored by the existence of the resonant cavity in the material given by the reflections on the sides of the device.
As the mirroring of one side is thinner than the other, the thinner "escapes" the LASER beam to the external environment. In Figure 10 we show the different stages of the emission of a semiconductor LASER.
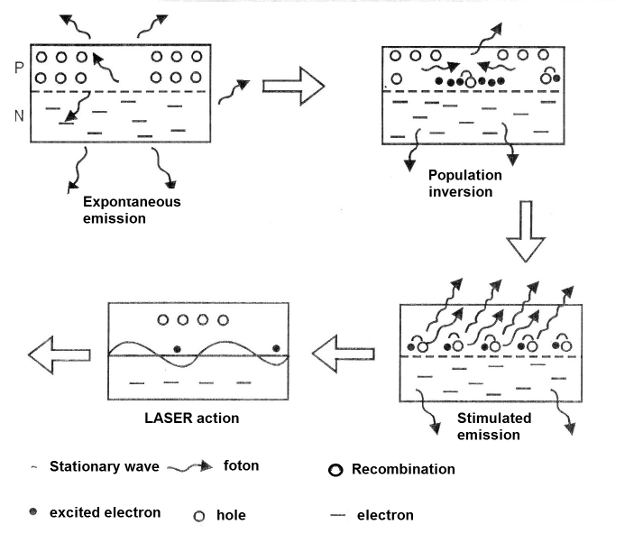
Although LASERS of this type usually operate with small currents, the driven pulses must have strong currents for population inversion, reaching in some cases densities of 100,000 amperes per square centimeter in the older types.
Current types can operate with smaller currents and even continuously, being used in many important applications and even have reduced prices.
APPLICATIONS
The possibility of obtaining extremely thin light beams, besides the fact of obtaining a monochromatic light, takes the semiconductor LASER to an infinity of practical applications.
Besides those that are present in equipment, but that we cannot see, as in the case of CD-ROMs and CD-players, we can mention many others.
LASER Pointers are simple "pens" which emit a narrow beam of light to produce an arrow or mark to point things on a blackboard or other location are examples of practical recreational applications of an LASER.
We can also mention alarms of passage with great rays of action, electronic sights, measurement devices, aligners of direction for vehicles, decorative effects in parties, light of point and many others.
BUILDING A LASER POINTER
In Figure 11 we show how to build a simple LASER Pointer with the DL660 using two common batteries and a pressure switch.
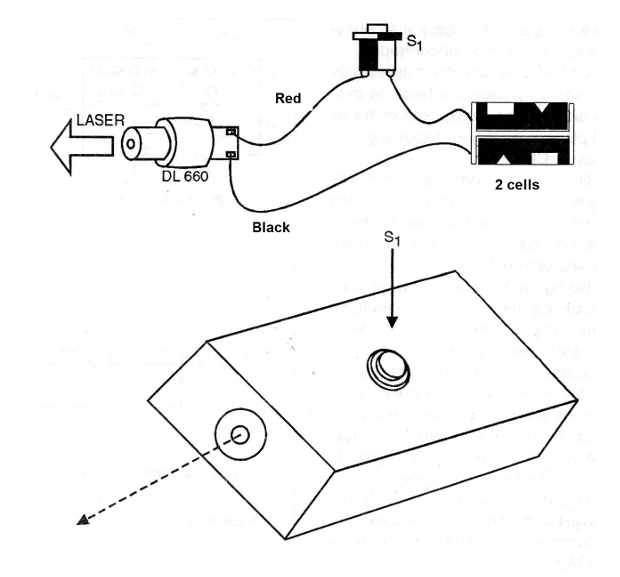
The beam with good collimation produced by this LASER allows to illuminate easily objects at distances of more than 100 meters at night.
SEMICONDUCTOR LASER DL660.
This is the DL660 semiconductor LASER that emits visible red radiation of 6,600 angstroms.
This LASER can be powered by batteries or sources (voltages from 3 to 6 V) generating a LASER with power of up to 4 mW with a maximum current of 26 mA.
The device already comes in an enclosure with the driven circuit, which makes its use extremely simple: just connect the red wire to the positive supply and the black to the negative so that the LASER beam is produced immediately.
Revised 2017